One does not simply hold a cloud of 100-million-degree plasma in one's coffee mug. Believe it or not, this is a fundamental problem of the ongoing quest for fusion energy, a theoretical solution to humankind's all-consuming passion for things that go brrrrrr. The process of fusion—the cramming together of two atomic nuclei of one element such that they form an atomic nucleus of another, heavier element—is capable of producing enough energy to, for example, warm an entire solar system, but requires some pretty extraordinary environmental conditions. Like those found in the interior of the literal Sun. Your cherished "World's Best Dad" mug has held some serious hell-brews over its celebrated history, but it simply will not contain star juice. No physical substance on Earth can manage it.
What's needed is magnets. Where your various materials will melt and burn instantaneously when they come into contact with a plasma burning at 100 million degrees, a magnetic field has no matter, and therefore nothing to burn, and a sufficiently strong one is capable of holding a blob of ignited fusion fuel in a relatively serene state of suspension. Fusion scientists call this process magnetic confinement fusion, and the ultra-bitchin' device they use to carry out their experiments in magnetic confinement fusion is called the tokamak. There are operating tokamaks all over the world, all of them pursuing their own courses of research (there's even a delightful tokamak video game), but of particular interest to humankind's energy needs is the quest for breakeven, the point when the plasma burning inside the tokamak produces more fusion energy than it requires in thermal input. Surplus energy from a fusion reactor could be used to power my electric shaver, and your ceiling fan, and eventually all the various gizmos that define modern life, all at once, potentially reducing and possibly even eliminating our reliance on polluting and planet-warming fossil fuels.
We are not talking about refrigerator magnets, here. A magnet that can suspend The Breath Of God harmlessly in a little floating cloud inside a little donut-shaped room has to have some seriously far-out, ahh, science properties. Normally these are gigantic electromagnets cooled to unfathomably low, deep-space-like temperatures, but even these cutting-edge big magnets are producing burning plasmas that come up tantalizingly short of breakeven. A magnet type capable of cranking out the outrageously powerful fields needed to squeeze and agitate and in all ways support a plasma capable of a self-sustaining, energy positive fusion reaction has, for many years of research, been out of reach.
That is, perhaps, until September of 2021. Researchers with Commonwealth Fusion Systems and MIT announced on Sept. 8 that their brand new prototype large high-temperature superconducting electromagnet, purpose-built using an all new manufacturing process, is capable of producing a magnetic field with a strength of 20 tesla, "the most powerful magnetic field of its kind ever created on Earth." The people involved in this project consider the successful production of a magnetic field of this strength a major scientific breakthrough. In their announcement of the successful field test, MIT described electromagnet performance as "the greatest technological hurdle" to constructing a tokamak capable of producing net energy gain, and CFS head of operations Joy Dunn confirmed to Defector Monday that the very next milestone, now that Big Magnet has produced Big Field, is the construction of an operational SPARC tokamak, the very design that once completed is expected to achieve the elusive goal of breakeven. There are no more parts to be tested or theoretical issues to be untangled! It's all nuts and bolts now. Simply manufacture the pieces, screw them shits together, flick the switch, and tune that sucker for breakeven, is what I'm hearing.
When a plasma scientist hears of a new electromagnet capable of producing the most powerful magnetic field ever created, they imagine all its incredible uses in the field of fusion science. But while I will certainly be happy to one day soon have an efficient and environmentally friendly local tokamak powering my Xbox, when you or I (or really any normal, perfectly sane person) learn of such a magnet, naturally we are first concerned with what would happen if we filled our bellies with metal ball bearings and then stood with our arms spread to the heavens as that unfathomably powerful magnetic field was switched on around us. Truly this is where the rubber hits the road. Wouldn't our torsos explode in a jaw-droppingly violent extravaganza of gore? Would our many giblets fly all over and paint the walls red and require a world-historically unpleasant shop-vac clean-up job? Talk about your scientific breakthroughs.
For help answering this important question, I put it directly to a physicist with MIT, who replied with, "This is an excellent question," but offered no answers and quite possibly has blocked my phone number. Buoyed by this encouragement, I reached out to Joy Dunn herself, who's been supervising the Big Magnet project just about every step of the way, and therefore clearly has nothing better to do than entertain questions about the blogger-annihilating properties of her potentially humanity-saving magnets. For two years prior to taking over operations, Dunn was head of manufacturing for CFS, and was on the actual damn production floor, "putting bolts in, turning wrenches," when the large magnet was built. Dunn was on the scene a month later when they plugged that sucker in and hit the switch and successfully generated that big fuckin' field. This is a person who understands the powers of Big Hot Magnet.
Dunn was kind enough to give Defector several otherwise extremely valuable moments of her workday in order to help explain, in layman's terms, what is going on here. This interview has been lightly edited for length and clarity:
So I see that the magnetic field produced by the big magnet is 20 tesla. Can you possibly explain what the hell that means?
Yeah, it means it's a very, very strong magnet. You know, to put it in context, your typical fridge magnet is about .001 tesla, so this is significantly stronger than your fridge magnet. And then a typical MRI machine operates at 1.5 tesla. So we're more than 12 times stronger than that.
And this big strong magnet is for SPARC? Or ARC? One of those tokamaks?
I mean, kind of all of the above. So this demo magnet proves the core concept that we can build a magnet up to 20 tesla, which is the magnetic field strength we need to confine and condense the plasma and get to net energy. So we'll scale up this technology, from the demo magnet to SPARC and then to ARC, but the core technology is the same.
But in the tokamak design there will be 18 of these big magnets?
Mm-hmm.
To a non-scientist that just sounds extremely bitchin': 18 big magnets in a big ring, each pumping out 20 tesla or whatever. Whoa! Will there be a lot of fine-tuning of the magnetic field to get the shape right? This is heavy-duty science stuff!
Exactly. These new magnets are called toroidal field magnets, so they're shaped like the capital letter D, and their job is to confine and constrict the plasma. That gets you the doughnut shape of your tokamak. And then there's two other magnet types: The central solenoid, which goes down the middle of the donut; and then the poloidal field magnets go around the poles, and the two of them combined then shape and move the plasma around inside the donut shape.
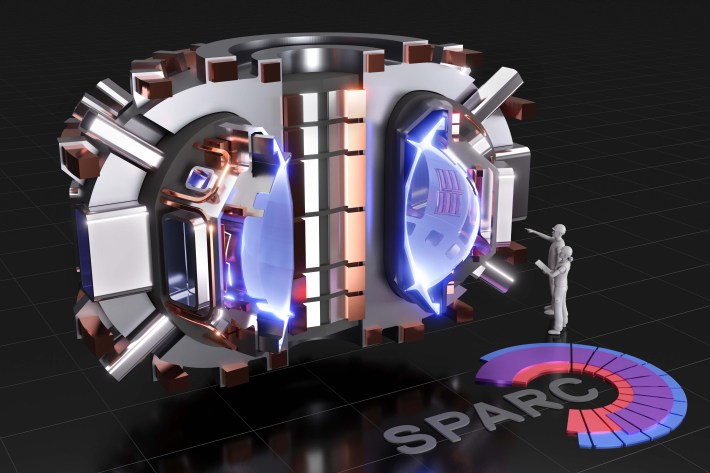
There's solenoid magnets inside like an electric transformer, yeah? That's everything that I know about solenoids and transformers and electricity, so I hope that's correct.
Yeah, so really similar to the transformer, it's able to pulse. You'll pulse the central solenoid on and off, and in basic terms that will give you your plasma shots, to get to that net fusion energy.
And these Big Magnets are high-temperature magnets? What kind of sick temperatures are we talking about there? Hit me with the high temps.
These magnets operate at about 20 kelvin, which is roughly 30 degrees above absolute zero. So it's about, like, minus-420 degrees Fahrenheit, roughly.
Wait.
Yes! That temperature actually makes them "high-temperature" superconductors, or HTS for short. Typically, the way that tokamaks have been built in the past is with LTS, or low-temperature superconductors. Those operate at four kelvin [Ed. note: That's minus-452 degrees Fahrenheit].
But these are both terrifyingly low temperatures!
Well, the difference in designing a system at four kelvin versus 20 kelvin is massive. Twenty kelvin affords us a less complex cooling system and makes our lives and operations much easier, just generally much easier to operate. And the cool part too is that with HTS we can actually test the magnet and test the layers of the magnets, which we call pancakes, at 77 kelvin, which is liquid nitrogen temperatures. And liquid nitrogen is easy to buy and much easier to operate, and gives us a really good check on how well the magnets perform before we get them very cold.
Yes, because as I am always saying, liquid nitrogen is not very cold. How do you make the big magnet colder than liquid nitrogen temperatures?
Well, first you reduce the temperature down to 77 kelvin with liquid nitrogen. So that's typically run in what's called a cryostat. It's a giant metal bathtub that the magnet sits in. And then from there we flow helium through the magnet, and that helium is cooled down to 20 kelvin, and lowers the temperature of the magnet.
When I think of a magnet, I think of a little horseshoe-shaped thing that Wile E. Coyote pulls out of an ACME box, or like a little Mickey Mouse–shaped guy that I use to stick the grocery list to the refrigerator. And they, like, uhhh, attract or oppose metal things? Do these big magnets work by opposing each other? And squeezing the plasma between their opposing fields? Or is that complete gibberish?
So the type of magnets that you're thinking of are a permanent magnet, that means that they're always on and they're gonna, you know, stick to your fridge, or something like that. The magnets we're building are electromagnets: You flow current through them, and that's how they turn on. The way that the toroidal field magnets are designed is that the magnetic field is in the center of those magnets. It's easier to visualize if you think of the interior space of the D shape. It's not that they're opposing each other, it's more that they're actually confining and constricting the plasma within the magnet itself.
So if I had a refrigerator nearby, it would not stick to the magnet?
Well, no. So most of the magnetic field is contained in what we call the inner bore, or the inner diameter of the magnet. So if your fridge was inside your magnet, it would actually be kind of suspended in the middle of it. Also, the magnetic field drops off really quickly with distance away from the magnet. Like, if you had a fridge that was very, very close, you know, you could end up sucking the refrigerator in.
But if you had a refrigerator stationed inside the tokamak, nothing. Right? Like, that would be too disruptive of the field?
Right, you couldn't turn the tokamak on if there was a fridge in the middle.
Honestly that's a little bit heartbreaking, to me.
No, but it's cooler than a refrigerator magnet! Because you can turn it on and off!
I have to say, I am having a hard time getting too excited about a magnet that has an on-off switch, if it can't even pin an overdue bill to a refrigerator.
OK, sure, but did you see the latest Fast and Furious movie? Not the least bit scientifically accurate, but they had electromagnets that they pulsed on and off and pulled cars all over and all sorts of cool stuff!
But just to be clear, moving fancy cars around is not something I could do with your big magnets? Like, what if 20 Defector bloggers charged your big magnet in 20 Tesla cars. Seems like you're saying we would achieve victory, in this scenario.
[in the manner of one defeated by facts and logic] Well, yes. I mean, it would depend on exactly how close you get to the magnet. But unless your 20 Teslas are actually inside the, you know, the inner bore of our magnet, you would probably win this one.
Ah, but could it yank metal fillings out of someone's teeth? Or what about this: What if I ate two big fistfuls of metal ball bearings, and then turned on the big magnet? Please don't say that this would not end in a horror show of explosive bodily dismemberment.
Well, unless you're standing right in the middle of it, probably not.
But if I were standing in the middle of it!
If you were standing right in the middle of the magnet, then yes, I think it would rip the ball bearings from your body and leave a big mess for us to clean up.